Product structural design refers to the process of designing the physical aspects of products, such as digital devices e home appliances, after their external appearance has been conceptualized.
How to understand the definition of “Product Structural Design”
This design phase bridges the gap between the aesthetic design e o processo de fabrico. For instance, in the case of an electric egg beater, once the external design detailing its shape and color is finalized, the next step involves structural design.
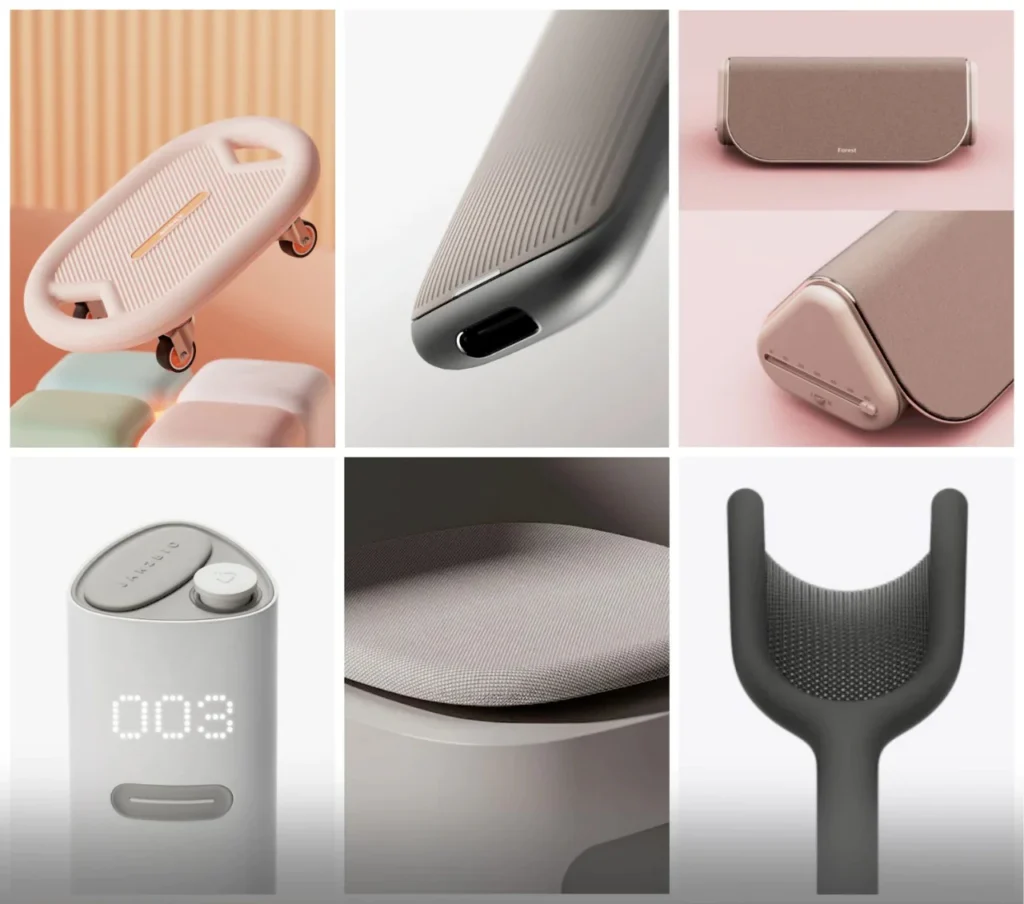
This includes using 3D modeling to incorporate internal components like the motor, circuits, and switches into the design. The process extends to dividing the outer shell into parts, creating molds for injection molding, and ensuring the compatibility and fixation of shell parts with internal components. It also involves considering the manufacturing processes and assembly requirements to design the unseen parts of the product.
Finally, the shell is produced through moldagem por injeção and assembled with the internal components to complete the electric egg beater.
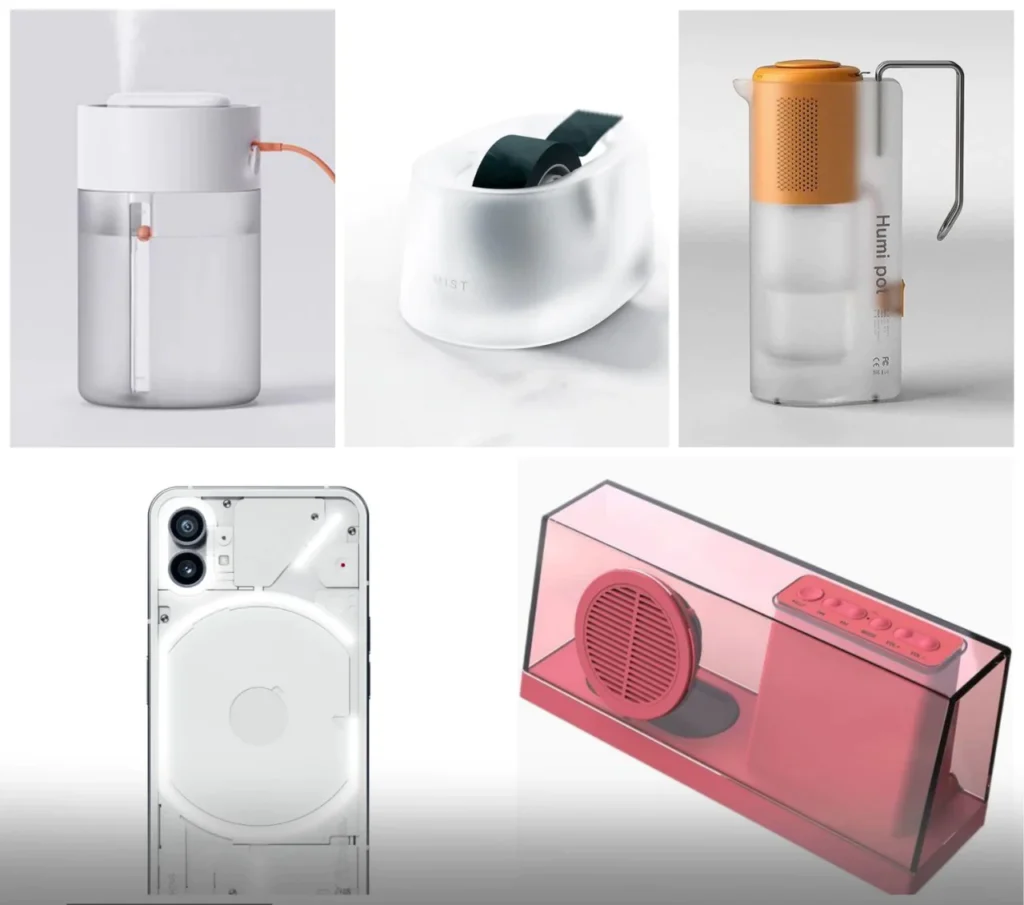
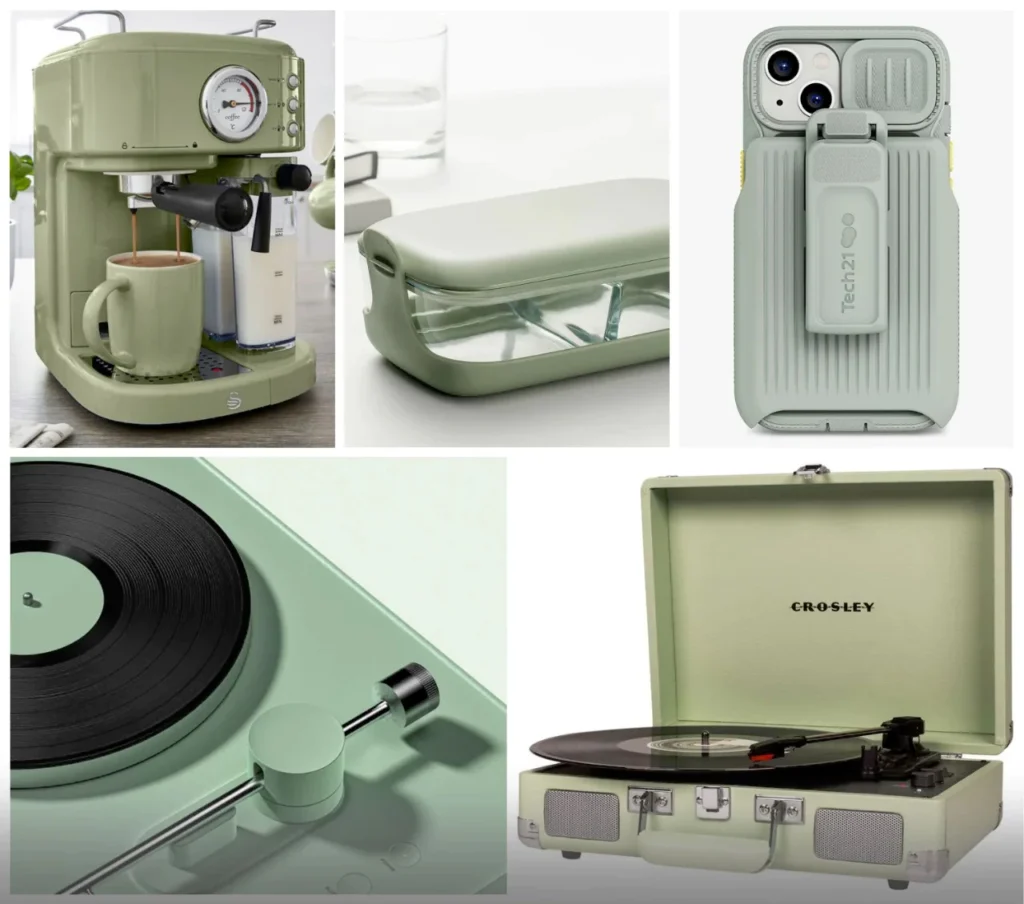
Product structural design is a comprehensive field that connects design with practical manufacturing. It encompasses a wide range of knowledge, including understanding of processes, parts, assembly, mold injection, functional testing, and project management.
Becoming a Product Structural Engineer: Basic Knowledge and Training
To become a product structural engineer, one must start with certain foundational knowledge. This section addresses the basics that a novice needs to evolve into a junior structural engineer, though having additional knowledge is always beneficial.
1. Mechanical Drafting
One of the fundamental requirements for product structural design is mechanical drafting, often learned during university studies. The necessary skills are basic and do not require manual drawing abilities or an understanding of various line thicknesses used in drafting standards.
However, it is essential to comprehend orthographic projections, side views, and basic concepts of first and third angles. Being able to interpret the dimensions and key features of parts from drawings is crucial. These skills form the foundation of product structural design.
Typically, students majoring in industrial design and mechanical engineering are taught this subject in college. If one did not grasp it well at the time, it’s advisable to revisit the textbooks, focusing on how several projection views represent a part’s shape.
Mechanical drafting is foundational for product structural design for two reasons.
First, the 3D software used in structural design, whether it’s CREO, SolidWorks, UG, CATIA, ou Fusion 360, operates on parametric design principles, transforming 2D sketches into 3D models. A lack of understanding in mechanical drafting makes learning these software programs challenging.
Second, structural design often involves interpreting numerous 2D drawings, such as part diagrams and specifications, to create 3D models. Without a basic understanding of mechanical drafting, it’s impossible to learn product structural design.
Dimensional Tolerances
Regarding dimensional tolerances, at the most basic level, it’s not necessary to understand complex concepts like the system of fits or tolerance zones. However, it’s crucial to know the meaning of the small numbers that follow the basic dimensions.
Dimensional tolerances are critical in ensuring the parts fit together correctly in the final product. While a deep understanding of advanced tolerance systems isn’t required at the beginner level, knowing the significance of the numbers following the main dimensions is essential. These numbers indicate the allowable variation in the part’s size, which is crucial for ensuring compatibility and functionality in the final assembly.
2. Software Learning
After grasping the basics of mechanical drafting, the next step is to familiarize yourself with CAD (Computer-Aided Design) software. At this stage, it’s not about memorizing shortcuts or complex operations, but rather about understanding how to open and modify drawings, add lines, and dimensions. This knowledge lays the groundwork for later learning 3D software, enabling the conversion of 3D part drawings into 2D manufacturing files and reinforcing the mechanical drafting skills recently acquired.
It’s important to note that pure 2D drafting is becoming less common in practical product structural design. However, mastering it can be beneficial, but remember, this is just the foundation for becoming a product structural engineer, not the entirety of the required skill set.
In modern product structural design, proficiency in 3D software is non-negotiable. Don’t be swayed by veteran engineers who reminisce about their prowess in 2D drafting or manual drawing. Engineering is not about craftsmanship alone; it’s about continually updating knowledge and skills. The efficiency gains from 3D software in product structural design are significant and cannot be matched by traditional manual drafting skills. A product structural engineer must be willing to continuously learn and adapt to new knowledge.
Choosing the Right 3D Software
The choice of 3D software depends on the industry you’re entering. For instance, the aerospace and automotive industries often prefer CATIA, despite some users favoring UG. In these industries, not knowing CATIA can be a disadvantage. UG is widely used in the mold and manufacturing industries due to its robust features. CREO, dominant in the home appliance and digital product design sectors, owes its popularity to early adoption by Taiwanese companies. SolidWorks excels in mechanical design, particularly in automation, due to its optimized features. Emerging software like Spaceclaim and Fusion 360 are also worth exploring.
It’s crucial not to become complacent with just one software. Different software has its strengths, and they are continuously evolving and borrowing features from each other. Believing that the software you learned is superior can hinder your growth as a designer and may lead to obsolescence. An open mindset and continuous learning are key.
Learning Resources for Software
Once you’ve chosen the software that aligns with your career path, there are numerous resources available for learning, including textbooks and online videos. The abundance of material online and in bookstores makes self-teaching a viable option.
Many people, after mastering software commands, find themselves still unable to effectively engage in product structural design. This is because software is merely a tool; the essence of product structural design lies in the knowledge behind it. Understanding the principles, processes, and intricacies of design is what truly enables one to excel in this field.
3. Industry Product Knowledge
When we talk about industry products in the context of structural design, we’re referring to tangible items that people use in their daily lives. This includes everything from mobile phones, cars, and toilets to toothbrushes and massagers. Essentially, any physical object that fulfills a user’s need or serves a specific function falls into this category.
The simplest products might be made from a single material and have a specific shape and function, like a stainless steel spoon. More complex products, however, consist of an outer shell and various internal components. Whether it’s a toilet brush or a rocket, the principle remains the same: a combination of an external casing and internal components, with the complexity varying based on the number of internal parts.
Understanding this, the role of product structural design becomes clear. It involves figuring out how to assemble the outer shell, how to integrate and secure internal components, and how to ensure these components deliver the desired functionality.
Deepening Knowledge On the Internet
To deepen your understanding, consider researching and analyzing the disassembly diagrams of common products. This will enhance your grasp of the concepts related to product shells and internal components.
The knowledge related to the product’s outer shell and internal components is a crucial aspect of product structural design. Each type of product has its unique features and challenges. It’s important to maintain a broad learning perspective and avoid becoming too narrowly focused on a single product type. Instead, aim to grasp the essence of each product category and engage in continuous learning.
Internal Components
Most products, except for those with a singular function and no internal components, contain core components like motors for power, heating elements for thermal energy, displays for interaction, control buttons, circuit boards, switches, connectors, dampers, seals, and energy storage units. These components are products in themselves, with their own sub-components.
For example, a motor might have coils, carbon brushes, magnets, shafts, and bushings. When these components are connected, they fulfill a specific function, like a heating plate connected to a circuit board and a socket in a rice cooker.
Some products have complex internal mechanisms with many moving parts, requiring knowledge of linkages, cam mechanisms, etc. This aspect of design is often handled by mechanical or automation engineers. Other products, like small appliances, might have fewer moving parts, focusing more on structural design.
The variety of internal components in different products is vast, and no single person can know them all. This diversity is why different fields can seem so distinct. For instance, an engineer experienced in designing smartphones might not be familiar with the heating elements in rice cookers.
Knowledge of internal components is accumulated over time. Being observant and curious about different components you encounter in everyday life can significantly enhance your understanding and expertise in product structural design.
Shells in Product Structural Design
In product structural design, the design of the product shell involves various manufacturing and surface treatment processes. The choice of materials and corresponding processing techniques is crucial in shaping the product’s external appearance and functionality.
Product shells are made from a variety of materials, each requiring different processing methods. Even with the same material, such as plastic, the processing technique can vary depending on the product’s size, shape, and other requirements. Common plastic processing methods include injection molding, vacuum forming, blow molding, rotational molding, and enameling.
Plastics in Product Design
The most common materials for product shells are plastics and metals. Within plastics, frequently used types include ABS, PC, PP, PE, AS, POM, and TPE. Each material has its own properties, molding conditions, and identification methods. Even within the same type of plastic, different brands or grades from various manufacturers can exhibit slight performance differences.
Metals in Product Design
The variety of metals used in product shells is also vast, including aluminum, stainless steel, galvanized iron, and cold-rolled steel. While these can be broadly categorized into aluminum, copper, and iron, the industrial development has led to a multitude of grades and types.
For instance, different aluminum grades are suited for different processes: 6063 is ideal for extrusion, ADC12 for aluminum die casting, 7000 series alloys are commonly used in aircraft and spacecraft, and 1145 is suitable for aluminum foil in food packaging. Understanding these nuances is crucial for effective product structural design.
A deep understanding of materials and their appropriate applications is essential in product structural design. Regularly collecting information and being observant about different materials and their uses will significantly enhance your ability to design effective and functional product structures.
4. Knowledge of various processes
Moldagem por injeção
Injection molding is the most common process for plastic components, especially in the design of household appliances. Simply put, injection molding involves melting plastic into a liquid state and pouring it into a metal cavity. Upon cooling, the plastic solidifies into the shape of the cavity. Understanding injection molding requires knowledge in three key areas: melting the plastic (understanding the physical properties of plastics), molding in the metal cavity (knowing the characteristics of plastics during molding), and ejecting the plastic part from the cavity (understanding the basics of the mold).
The most critical aspect related to product structural design is the ejection of the part. To remove the plastic part, the metal cavity, or mold, must be able to open and separate into two halves. If the shape of the cooled plastic part gets stuck in the cavity, it cannot be removed. Therefore, the angle between the plastic part’s shape and the mold’s parting line must be less than 90 degrees to prevent this issue.
Mold design can include movable sections to facilitate part removal. For example, a plastic bowl can be removed by first moving the outer metal layer that holds it. These movable parts in molds, known as sliders ou lifters, make the design of plastic parts more flexible. However, their use should be minimized as they can increase mold costs and complexity.
Other Plastic Processing Techniques
Other techniques like blow molding, enamelinge rotational molding each have their unique processes and implications for product structural design. It’s beneficial to understand these different techniques to broaden your design capabilities and options.
5. Assembly Design in Product Structural Engineering
After understanding the basic structure of various products and their molding processes, the next crucial aspect is the design of product assembly. This is a key component of product structural design, involving the logical arrangement and secure attachment of internal components within the product’s external shell.
Internal Component Assembly
O assembly of internal components is not just about placing them inside the shell; they need to be precisely positioned and securely fixed to function effectively. In product structural design, consider these aspects: positioning of parts, securing them, protecting them, and ensuring their functionality. These considerations require a holistic approach and often need revisiting during the design process.
Positioning of Parts
Designing the exact location of each part within the product is critical. This involves determining their spatial position in three dimensions and ensuring the positioning method is practical and effective. Over-positioning and difficulty in positioning, especially considering the manufacturing tolerances of the parts, are common challenges. Even experienced engineers can make mistakes in this area, such as relying on hole positioning without considering the achievable precision in manufacturing. Understanding the concept of degrees of freedom, a topic covered in mechanical design courses, is essential here.
Securing Internal Components
Differentiating between positioning and securing components is vital. Avoid using the same structural feature for both positioning and securing, especially when high precision is required. Positioning demands precision, while securing requires strength. For instance, using screw posts for positioning might work in low-precision scenarios, but for high-precision requirements, dedicated positioning posts with tighter tolerances are necessary, with screws serving only to secure the parts.
Common methods for securing parts include screwing, gluing, welding, riveting, and sometimes snap-fit mechanisms, though the latter can be less effective against impacts. The challenge lies in ensuring adequate strength, and learning from existing designs can be highly beneficial. Further, revisiting university-level mechanics, material science, and finite element analysis is crucial. Modern simulation software, capable of modeling scenarios like drop tests, is a valuable tool for young engineers to surpass traditional experience-based methods.
For specific details on snap-fits, screw posts, ultrasonic welding, riveting, and adhesive bonding, specialized resources should be consulted. It’s important to emphasize the significance of learning finite element analysis software and staying updated with the latest manufacturing capabilities. Relying solely on design experience without adapting to new technologies and methods can quickly lead to obsolescence in this rapidly evolving field.
6. Protecting Components in Product Structural Design
In product structural design, protecting the internal components is a critical focus. Different products operating under various conditions require specific protection for their internal parts. The most common requirement is strength; it’s imperative that components do not dislodge or break during normal or extreme conditions, such as falls or during transportation. The structural design must ensure durability for regular use and resilience against extreme situations. Different products have different quality standards and, consequently, varying strength requirements.
Common protection needs include cushioning and shock absorption (e.g., for motors), as well as thermal insulation, dustproofing, and waterproofing. These measures are essential to extend the product’s lifespan and ensure its reliable operation. The specific learning content should be tailored to the particular product and its components.
Waterproofing and Dustproofing
Waterproofing and dustproofing are often specified by IP (Ingress Protection) ratings, such as IP65, which indicates complete dust resistance and protection against water jets. To achieve this, product structural designs commonly incorporate silicone sealing rings, potting compounds, and design angles for rain protection.
Thermal Protection
Thermal protection of internal components is another critical consideration in product structural design. Components like heating elements, motors, and circuit boards generate significant heat during operation. If this heat is not adequately dissipated, it can damage the components.
Common solutions include adding cooling fans, heat sinks, thermally conductive silicone, and graphene-based heat dissipation materials. Thermal analysis knowledge and software are used to calculate whether a particular heat sink can meet the cooling requirements of the product components. The principles involved include heat transfer, convection, and specific heat capacity, as taught in high school physics, but practical application in product design requires professional knowledge.
Different products and components require varied protective strategies in structural design. As a product structural designer, it’s essential to accumulate a wide range of experiences. Learning from successful designs in one product category and applying those insights to others can elevate your design skills above industry peers.
7. Functional Implementation
In product structural design, considering the functional implementation of components is a specialized and detailed aspect of the design process.
For instance, in electronics, the basic function of a speaker involves creating openings for sound to pass through. A more advanced understanding includes adding sealing foam between the speaker and the casing to form a front sound chamber, and ensuring the area of the sound holes meets the speaker’s specifications for optimal sound transmission.
In high-level design, the creation of a sealed rear sound chamber is considered, where the volume and shape are specifically designed to meet the speaker’s acoustic requirements, ensuring optimal f0 and sound frequency curves for superior sound quality. This aspect of design, often invisible to outsiders, involves significant work and detail. Learning in this area requires a deep dive into each functional component, understanding, designing, testing, and improving them.
Designing for Movement and Space
Another common aspect of functional implementation is designing for movement and space. For example, when a motor drives a linkage mechanism, the structural design must consider the mechanics of the linkage’s movement and ensure adequate space for its operation. Since most products aim for compactness, internal space is often limited. Determining appropriate clearances and understanding the minimum space requirements for each type of functional component is crucial and comes with experience.
Overcoming Industry Barriers in Design
The knowledge related to protecting internal components and achieving their functionality often forms the main barrier to entry in different industry sectors of product structural design. However, these challenges are not insurmountable. They involve understanding how to use each component safely and effectively, following a unified thought process, and supplementing knowledge in unfamiliar areas. Much of this knowledge has been distilled into industry-specific experiential values.
While the underlying theoretical reasoning can be complex, in practice, understanding these experiential values can lead to rapid and effective product structural designs. It’s important not to be intimidated by seemingly complex theories. Believing in the versatility and capability of a product structural designer is key to success in this field.
The Role of Experience and Continuous Learning in Product Structural Design
The previous discussion on product structural design highlights the importance of experience values. It might seem that mastering software, understanding basic product concepts, and accessing design experience values are sufficient for product structural design. This is both true and untrue. For many junior product structural designers, this is indeed how they start. However, the correct interpretation and application of these experience values require deeper internalization and understanding. Knowing the principles of success but failing to apply them effectively is a common pitfall.
The Need for Updated Knowledge and Skills
In product structural design, when reconstructing appearance models, knowledge of manufacturing processes is crucial. For example, understanding whether a design is moldable and how to optimize it is essential. This knowledge needs to be constantly updated and refined. In preliminary structural design, considerations include how to facilitate assembly and part production, improve production efficiency, and understand assembly-related issues. These aspects also require continuous learning and updating.
When refining product structural designs, minor adjustments that might seem insignificant to an outsider can be crucial. Decisions about the appropriate amount of snap-fit engagement, the right clearance between parts, or the optimal dimensions for ribs in injection molding are all based on deep understanding. This understanding must be thorough, encompassing both the how and the why.
Motorola’s Design Guidelines
For instance, Motorola’s design manual suggests a 0.075mm gap between the lens (transparent window panel) and the surrounding shell. A junior designer might simply follow this guideline, but a more experienced designer will delve deeper.
This specific value relates to the CPK values of injection-molded shells and the precision of lens cutting. It involves understanding statistical tolerances and requires continuous updating of knowledge. In a small-scale factory setting, a 0.075mm gap might not be appropriate due to different CPK values compared to Motorola’s supplier system. Recognizing these nuances is what distinguishes a master designer who can adapt to different environments and industries without issue.
To excel in product structural design, one must engage in ongoing learning and skill updating. This approach ensures that a designer remains versatile and effective, regardless of changes in location or industry. It’s not just about knowing the rules but understanding their underlying reasons and adapting them to different contexts.
Other Knowledge
Mastering software, understanding principles, and gaining experience in product structural design are just the beginning. A product structural designer’s role encompasses much more.
Knowledge of Components
Firstly, a structural designer needs to be knowledgeable about various components. Many products are designed from the inside out, starting with selecting internal components, arranging them effectively, and then designing the exterior. This requires choosing appropriate components and placing them in positions that facilitate both functional and aesthetic design.
This task involves understanding the working principles of these components and collaborating with industrial designers and hardware engineers to meet both functional and aesthetic requirements. Due to the complexity of this work, specialized roles like Stack Engineers or Product Architects have emerged, requiring a blend of product thinking, structural thinking, and design aesthetics, along with hardware knowledge.
Factory Visits and Understanding Manufacturing Processes
A product structural designer must also visit various manufacturing and assembly facilities, such as mold factories and production lines. Understanding how different designs perform under various manufacturing and assembly processes is crucial.
This knowledge allows the designer to optimize their designs for manufacturability, improving part processing yield and assembly efficiency. Structural engineers are responsible for creating initial assembly instructions, which serve as a guide for production line setup and product understanding.
Furthermore, a product structural designer is responsible for organizing the entire product’s Bill of Materials (BOM) and distributing the corresponding manufacturing drawings to various suppliers, including mold makers, sheet metal factories, stamping factories, silicone factories, etc.
Coordinating with these suppliers to ensure each part is produced correctly and meets quality standards is a significant part of the job. Decisions about acceptable tolerances and surface flatness for assembly compatibility are crucial and fall under the designer’s purview. Due to the workload, specialized roles like Mold Engineers have been developed to handle these specific tasks.
The Ongoing Responsibilities of a Product Structural Designer
The job of a product structural designer doesn’t end with the completion of designs and the initial assembly of the product. In fact, a new and often longer phase begins, focusing on quality assurance and compliance with standards.
Before a product hits the market, it must pass various quality checks and certifications, such as 3C standards domestically or FDA and CE standards for exports. The designer’s role includes researching these standards beforehand to ensure compliance, analyzing issues post-testing, and iterating the design until it meets all requirements. This cycle of assembly, testing, problem-solving, and retesting continues until the product is ready for mass production.
Even when a product is ready for mass production, the structural designer’s work isn’t finished. They must ensure that every part meets the required standards and communicate these specifications to each supplier. This involves defining acceptable tolerances and creating traceable documentation to address any quality issues during mass production. In case of problems, the designer must devise both immediate and long-term solutions, effectively acting as a “product caretaker.”
Recap: Knowledge and Interactions in Product Structural Design
Reflecting on the foundational knowledge required for product structural design, it’s clear that a solid understanding of design principles, software, component knowledge, manufacturing processes, assembly techniques, quality standards, and problem-solving logic is essential.
Interactions with various professionals are a constant in this role. A product structural designer collaborates with product planners, industrial designers, hardware engineers, suppliers, production line staff, quality control personnel, testers, and project managers.
A comprehensive understanding is necessary, encompassing aesthetics, hardware, product planning, coordination with various suppliers and assembly plants, knowledge of quality standards, and familiarity with testing methods. The ability to identify and solve problems is crucial in this multifaceted role.
Conclusion: The Versatile Career Path of a Product Structural Designer
A product structural designer is intricately involved in almost every stage of a product’s lifecycle. Their role is not confined to the initial design phase but extends through the entire process of bringing a product to market. This comprehensive involvement provides them with a unique set of skills and knowledge that makes them highly adaptable and versatile in their career paths.
Potential Career Transitions
Due to their extensive experience and understanding of various aspects of desenvolvimento de produtos, product structural designers often find opportunities for career transitions within and outside their original field. Some common career shifts include:
- Product Planning: Their deep involvement in the design process equips them with the skills to strategize and plan new products effectively.
- Procurement and Resource Development: Understanding supplier dynamics and manufacturing processes can lead them to roles in procurement and resource management.
- Quality Planning: Knowledge of quality standards and testing procedures can pave the way for a career in quality assurance and control.
- Factory Management: Frequent interactions with production lines and manufacturing processes can lead to roles in factory management.
- Project Management: Exposure to the entire product development cycle, from conception to production, makes them suitable for project management roles.
The only fields where transitions are less common are industrial design and hardware engineering, which require specialized skills that are not typically the focus of a product structural designer.
A World of Opportunities
In summary, the career of a product structural designer is marked by a breadth of knowledge and a depth of experience that opens up numerous pathways for professional growth and transition. Their comprehensive understanding of the product lifecycle, from design to production and quality assurance, makes them valuable assets in various roles across industries.